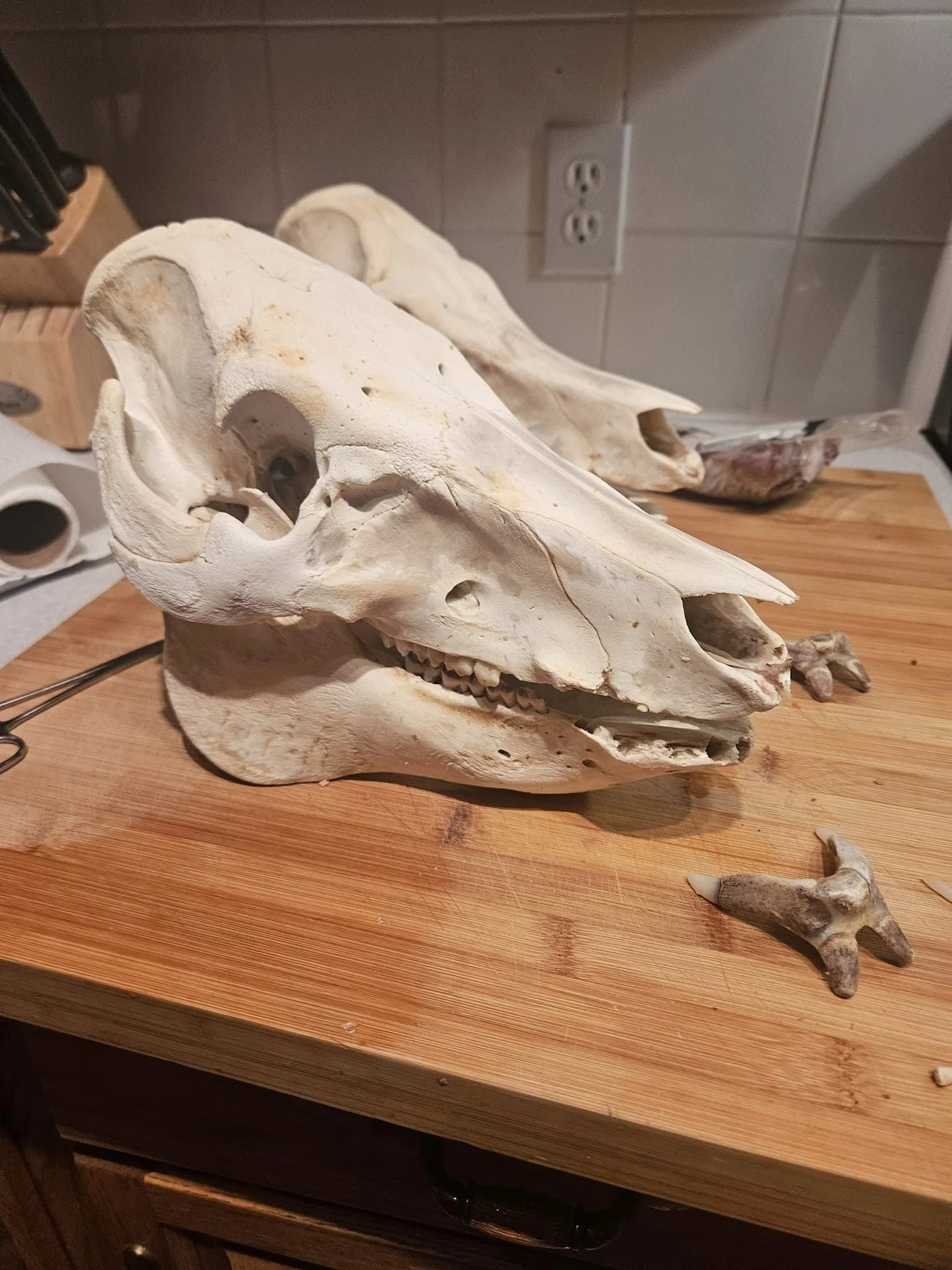
BONE DEGREASING INFORMATION
Why degrease bone?
Bones have grease housed in several places, including the cancellous bone in the interior of most bones. This is often a combination of organic materials including, but not limited to, marrow and other fatty tissues. These tissues break down and leave behind grease, which seeps through the porous outer cortical bone. This fat can become rancid and odorous, unappealing to look at and create a surface which dust sticks to. It is therefore favorable to degrease bone, except in cases where DNA procurement is foreseen.
There are six potential parts to degreasing a skull which may be useful. These are saponifiers, surfactants, oxidizers, enzymes, water and heat. Each is useful and may come with detrimental trade-offs or require a separate method of degreasing. Proper degreasing methods are important, because improper degreasing can compromise collagen. Compromised collagen produces brittle or powdery bone.
Research is ongoing, and this will be updated semi-frequently. If you notice a mistake, please reach out! Collaboration can improve this free access document. Changelog and version located at the bottom of the document.
Introduction
Most professional and amateur skull cleaners understand the basics of degreasing bone, but few understand the chemistry behind it. Understanding “what goes on in the bucket” impacts efficacy, efficiency and quality of the final product. For safety and practicality reasons, only reasonable and cost effective chemicals will be discussed. The following information was assembled during the development of the product. The hope is that it brings organization to the cacophony of opinion and disinformation on the internet on the subject.
This is not a peer reviewed academic paper. When considering use, understand that these tests do not represent statistically rigorous samples. It is not recommended that anyone mix or use chemicals. This is for information purposes only. The author is not responsible for any problems, harm or damage you may encounter while using chemicals.
Degreasing Information
Composition of Bone
Before discussing bone degreasing, it is important to understand the components of bone and fat. Bone is made of a mineral called hydroxyapatite, which is calcium phosphate [Ca3(PO4)2]3Ca(OH)2. Collagen makes up a significant part of the bone matrix. Type 1 makes up to 90-95% of all collagen in bone (Eriksen, et al, 2009 and Shehata and Krap, 2024). By ratio, bone is about 70% inorganic, 5% water and 20-25% organics (Lambri et al, 2018). The hydroxyapatite can be thought of as a rigid and hard, yet brittle, scaffolding for the collagen which provides flexibility and tensile strength. Simply put, there are four relevant kinds of bone. For the purposes of this document, these are divided into two macroscopic types and two microscopic types. The two macroscopic types are cortical bone and cancellous bone. Cortical bone, which is dense, often thick, and found on the outside of most bones. Cancellous bone, which is found on the interior of bones and contains marrow. This is where much of the grease is found. The two microscopic types are woven bone and lamellar bone. These are separated by the orientation of collagen (Bosch et al., 2011). Woven bone has random collagen fibers and is disorganized. It contains more osteocytes and fewer lamellae. This is common in juveniles and fish. Lamellar bone, found in adult mammal bone, is more organized, contains more lamellae and parallel collagen fibers. It is stronger and more resistant to heat.
Most people understand that compromising the minerals in bone results in a poor product. Altering the collagen will change the bone mineral mechanics (Viguet-Carrin et al, 2005) possibly leading to brittle bone (Burton et al, 2014) or possibly flakey, powdery bone. This can occur chemically, enzymatically or with excess heat. Zdzieblik et al (2021) discuss the positive relationship between increased collagen peptides and bone mass in osteopenia and osteoporosis in postmenopausal women, further demonstrating the importance of this relationship. It is therefore imperative to shelter collagen as optimally as possible for the best results. For types of fat, how they break down and additional information on bone tissue please refer to Turner-Walker 2012.
When discussing problem areas such as the maxilla near the zygomatic joint or the dentary, it is likely that the grease itself isn’t much more resilient but that there is an increased volume of grease and dense bone is preventing degreasing agents from entering or products from leaving. This may not be the case if the skull has a thermal history causing crystallization of the triglycerides. It is possible that, with sufficient time and degreasing agents, the grease becomes entirely saponified. To test this, diabetes needles were used on a marten skull to access the dentary via the mental foramen and injected with degreaser. The maxillae were injected on the dorsal surface of the maxillary zygomatic process, directly into a pocket of “stubborn grease”. A tricky area around the basisphenoid was also injected. This was successful in rapidly breaking up these tough spots. It is unclear if this simply provided an entrance/exit, or if the direct application of degreasing agent made it successful. It seems reasonable to assume that it was probably a combination. This can be tested by using a needle to puncture a skull with no injection. Unfortunately, larger gauges of needle will be required in order to get through thicker bone on larger mammals which will leave a noticeable hole if either the tooth sockets or foramen aren’t used. This may be cosmetically repairable with minimal effort.
How does Grease Move?
Grease moves through bone via diffusion. Saponification, dissolution, heat, water movement and grease rendering all impact the speed at which diffusion works. In this case, the degreasing mixture, carried by water, moves towards the hypertonic grease through the bone until it reaches equilibrium. Once there, it breaks down the grease through its typical means and the products are exported through diffusion gradients, moving from areas of greater to areas of lesser concentration outside the bone. Grease itself may move through the bone too, especially when heated in soapy water, to areas of lesser concentration. To expedite degreasing, using fresh water with degreaser in it is important to keep diffusion gradients high. For these reasons, and because it is less likely to harm collagen, it may be reasonable to use lower percentages of degreasing material to save money and increased water change frequencies.
Water
Water is the most ubiquitous ingredient in degreasing. It is a polar covalent solvent. Almost all applications of degreasing will include water. It serves as a host or diluter for most degreasing agents, soaps, oxidizers and as a carrier of lifted or saponified grease. Typically grease is discarded with water changes as the grease carrying capacity of water reaches its limit, the degreasing agent runs out or both. This can vary in timeframe depending on the amount of grease, heat of solution and strength of degreaser.
Many skull enthusiasts do not realize that long term water exposure can harm bone. This happens through several mechanisms which includes, among other things, bacterial action rotting the collagen, chelation of minerals from the skull, and carbon dioxide interacting with the water to form localized carbonic acid which then dissolves the bone. It is therefore useful to find ways to limit the time a skull is submerged.
Heat
Most skull enthusiasts understand that heat mobilizes or renders grease and therefore heat greatly impacts the answer to the a common question: How long to degrease a skull? Favorable temperatures vary based on animals, but typically are around 30 degrees Celsius or less for fish and bird and 44 Celsius or less for mammals. These temperatures for mammals allow for a few degrees of overages from heat controllers, ensuring heat does not exceed 46 degrees Celsius. This is because fats for many mammals melt around 40 degrees Celsius with various culinary references on the internet suggesting bovid fat renders at 55-60 degrees Celsius. Soap, a product of saponification, melts at around 55 degrees Celsius. Collagen denatures as low as body temperature (Leikina et al, 2002). This heat bracket may be most useful in specimens such as arctic fish. The most useful bracket for mammals is 46 degrees Celsius (Lambri et al, 2018) and shrinking in human pelvic elements occurs at 61 degrees Celsius (Danielsen et al, 1994), so a target safely below 46 degrees Celsius is ideal to allow for slight overages from the heaters. A temperature of 48 degrees Celsius is generally accepted as a safe temperature by bone cleaners and taxidermists, though this author has found 46 degrees Celsius to be favorable for not disarticulating the zygomatic joint. Lamellar collagen in bone is more resilient than collagen found in other tissue but there is no reason to use excessive heat when degreasing.
It is the understanding of this author that heat makes collagen triple helixes “bow out” in the middle. This leaves the fibrils more vulnerable to hydroxyl, methyl or other chemical insertion and enzymatic activity. This separation at the cross-links is apparently temporary, and, unless denaturation occurs, the triple helix structure will reform when cooled. This understanding is likely to be refined. It is shared to pass along the idea that heat may be harmful in ways other than denaturation.
Heat also increases the speed at which chemical reactions occur. While there are critical temperatures for some reactions that aren’t reasonably achievable because of the collagen heat limit, appropriate application of heat is critical in speeding up the chemical reaction and limiting exposure to water and other potentially harmful chemicals.
It is noted that some efforts have been made to accumulate fat rendering temperatures by several amateur authors. These temperatures are unreferenced and may not be accurate. As organ fat, back fat and other body-fat rendering temperatures may differ, these may not be laterally comparable to marrow-fat temperatures. This effort may also be futile because finding an uppermost temperature that doesn’t harm collagen is likely the best answer. This is because collagen presents a hard limit for heat, and using the highest possible heat maximally mobilizes the grease.
After testing on both dermestid and simmered skulls, cleaning with very low simmering (sub 60C) appears to provide superior degreasing times because significant degreasing occurs during simmering. It also is far more likely to cause damage to collagen because the margin for error is much tighter. Further excessive heat, such as 100C or greater, will cause loss of protein and “smoothing and softening of the bone” (Bosch et al, 2011). As the collagen is destroyed by heat, it may release CO2, HCO3 or Hydrogen ions. These interact with the hydroxyapatite via ion exchange, causing damage as seen in Bosch et al, 2011 and noted in White and Hannus, 1983.
Saponifiers
Saponification is the process by which fats are turned into soap. This is achieved by combining a base, such as ammonia, with triglycerides like animal fat. There are different strengths and kinds of saponifiers out there, each with advantages and disadvantages. These may be the most cost effective way of dealing with triglycerides but are also problematic for collagen.
Weak bases such as ammonia and soda ash are popular skull degreasers. These bases don’t completely disassociate in water and therefore may throttle the amount of hydroxide in the water at any given time. Unfortunately, other than soda ash, metasilicate and baking soda, other common weak bases smell horrible including ammonia. No matter what type of base is used, hydroxides will be released and saponify. It is possible that controlling the concentration of stronger bases such as lye can produce favorable results like ammonia, though mitigating other processes like oxidation and chelation is a consideration when ions are involved.
One has to consider cost, strength, availability and negative side effects. Because it is covalent and does not oxidize beyond the hydroxides, ammonia is heavily favored in the skull cleaning community despite being a weak saponifier.
Hydroxides (OH) form from every chemical with a pH higher than 7. This includes ammonia (NH3), lye (NaOH), and potash (KOH). The relative concentration of OH is how pH is measured “basic”. Hydroxides react with, or hydrolyze, grease (fats) breaking the ester bonds in the grease, turning them into soap and glycerol. This is called saponification. Glycerols are technically a solvent, but because they are polar and fats are non-polar, fats don’t readily dissolve in them. Hydroxides are known to damage collagen, so should be controlled. It is possible that throttling the concentration of stronger bases, simulating the trickling effect of hydroxides from weaker bases such as ammonia, may make them more viable as degreasers of bone.
Ammonia (NH3) Overwhelmingly the most popular saponifying degreaser of bone. Weak base. It is cheap and readily available. Despite these advantages, it is a poor degreaser. Costs escalate over time and longer times in solution may cause water to damage bone. It is very noxious.
Ammonia works by the following equilibrium reaction (Turner-Walker, 2012):
NH3 + H2O ↔ NH4+ + OH-
The pH of ammonia is approximately 12.5, and about 1% is hydrolyzed at any given time. The hydroxide ion and ammonium bind to some of the fatty acids to form ammonium salts and water. As this happens, more ammonia is hydrolyzed into ammonium and hydroxide, continuing the process until ammonia is used up or off-gassed completely. Importantly, hydroxide ions are known to cause damage to protein (collagen). As a result, ammonia does have bone damaging potential. This is usually negligible, unless the ammonia is overheated, the bone is overexposed, or the concentration is too high.
A common misconception persists in the skull cleaning community that ammonia and soap “cancel out”. Ammonia and soap function in two different and complimentary ways, meaning it is likely that it is more effective to combine them. Many commercially available soaps contain ammonia and cloudy ammonia contains soap. Ammonia breaks down soap scum, so will help remove any soap scum, which is just a deposit of calcium and soap.
Borax Creates a high pH environment and therefore likely saponifies somewhat. It breaks down into boric acid and sodium hydroxide and therefore isn’t ideal.
Potash and Lye Extremely strong degreasers. Easy to source, extreme handling concerns, storage and disposal concerns and noxious. Strong oxidizing agent that will destroy protein including collagen. Not recommended for cleaning bone. Common additives in industrial commercial degreasers in amounts of 0.5-1%.
Soda Ash is a popular hydroxide forming weak base.
Sodium Metasilicate (Also known as waterglass) This is the saponifier used in Bare Bones Solutions degreaser. It buffers at a pH of around 12.5.
When mixed with water hydrolysis occurs and produces orthosilicic acid and hydroxide (Jantschke et al 2014 and Zhang et al, 2015). A silicic acid simply contains a silica attached to an oxide and a hydroxyl group. Silica may play a role in biomineralization and metasilicates are being explored for novel positive interactions because living bone has receptors for the material (Vere et al, 2013). It has anti-corrosion properties of metals and non-metals, due to interactions with the silica. It is used as a bleach stabilizer, slowing rapid decomposition of peroxide and other bleaching agents. Metasilicate is used as a whitening agent in recycled fibers (van Heiningen et al, 1999) providing explanation for the white color of skulls after exposure to metasilicate mixes.
Trisodium Phosphate (TSP) Cheap, powerful degreaser that comes in a powder for cheaper shipping. Do not mix with ammonia because it forms ammonia gas on contact. This was the first substance tested by what would become Bare Bones Solutions. It produces an alkaline solution of pH greater than 11. It is available commercially in both chlorinated and unchlorinated forms. It is versatile, finding use as an anti-bacterial food additive, in stain removal, grease removal, anodizing and more. It is cheap, easy to source and handle, efficient, lacks noxious smell and shelf stable. This gives TSP great advantages as a bone degreaser, though it is too powerful and damages bone even at miniscule concentrations.
It is banned in a dozen states for causing trophallaxis in waterways, damages bone over time, and has a low thermal decomposition temperature (73.5C) which means degreasing must be done at a lower temperature. When heated, it can release toxic fumes. It is therefore ill-suited for bone degreasing.
The phosphate in TSP reacts negatively with proteins, including collagen. This is because it is an oxidizing agent. TSP will also react negatively with calcium in bone over time in a process called chelation. In this process, phosphate will bond with surface calcium from the bone to form aqueous apatite in solution.
Surfactants
Surfactants serve several roles, including as wetting agents, emulsifiers and solubizers. Wetting agents reduce the surface tension of water, allowing it to spread more easily and lift grease. Emulsifiers bind liquids that normally do not mix, creating an emulsification. This is achieved by dispersing one liquid as tiny droplets into the other. Non-polar solvents can be emulsified in water, which is highly polar, helping to bend the generalized rule that “like dissolves like”, or non-polar dissolves non-polar (as in triglycerides with non-polar solvents). Emulsifying a non-polar substance in water may have limited success, but some is better than none. Solubizers are used to make grease and other water-insolubles dissolve in water. This process is important, because it aids in mixing of water and grease which normally do not mix. It is accomplished by coating small parts of grease in hydrophilic micelles which then disperse and mix. In many cases, a surfactant will have several roles.
There are two primary types of surfactants available. These are ionic and non-ionic. Ionic surfactants are typically weaker but gentler on skin and eyes. Negatively charged, or anionic, surfactants may denature collagen in small amounts (Nandi et al, 1985). Positively charged, or cationic, surfactants do not appear to damage collagen. Non-ionic surfactants are stronger but harsher on skin and do not appear to damage collagen. They are found in many laundry detergents. Unfortunately, chlorine producing agents are also in many laundry detergents, so care must be taken when selecting a laundry detergent for use. Surfactants add an important element to the grease removal process, especially when used together with saponifiers.
Sodium Lauryl Sulfate and Laureth Sulfate Extremely successful and popular in bone degreasing dish soaps. Used in shampoos and dish soaps because they foam well. Anionic (negatively) charged surfactants that may denature collagen, even in small amounts (Nandi et al, 1985) but that may not apply to collagen bound in bone. Sulfates are very weak oxidizing agents.
Oxidizers
Several oxidizers have been popular in bone cleaning. They work by accepting electrons from another substance, causing the substance donating the electron to become oxidized. These include chlorine (bleach), peroxide and hydroxide (ammonia). Phosphates and sulphates can also be used. Along with the damage to proteins, some also accept calcium ions from the apatite of the bone in a process called chelation. Oxidizers damage proteins, including collagen in the bone. Chlorine has long term negative effects on bone, continuing to damage bone well after drying.
Hydrogen Peroxide is the most common oxidizer and likely the only devoted oxidizer encountered in skull cleaning. Used ubiquitously to whiten bone in the last stage of cleaning and often to quickly (and poorly) degrease skulls in European mounts. Known to damage bone by forming oxygen radicals in the water-peroxide reaction which then oxidize collagen. It comes in many forms including liquid developer, various percentages of hydrogen peroxide as well as oxyclean. It is often debated which product is superior, but ultimately what matters is percentage, heat applied and time of exposure. As with any chemical, too much heat or exposure has increasingly deleterious effects on the bone.
Solvents
Organic solvents appear to have the most success (Guilmnot et al, 2014), though they are volatile with low vapour pressures, expensive and are rapidly absorbed through skin (Boman and Maibach, 2000). Polar solvents will be miscible in water and may be less likely to dissolve triglycerides, but not always. Non-polar solvents will not be miscible in water and tend to dissolve triglycerides readily. Choosing the right solvent for the job can be difficult based on cost, disposal, volatility and even danger level.
According to Turner-Walker (2012), the most effective solvent cleaning was a combination of acetone, ethanol and water in a ratio of 1:1:1 with a few drops of a strong surfactant. They added some ammonia as well, which was said to greatly improve the test. Though effective, this may not be the most practical approach due to the volatility and cost of materials used. The following is a list of solvents that is not exhaustive. Many were excluded for lack of availability, extreme toxicity or other unreasonablities.
Acetone Fantastic degreaser. Moderately polar solvent. Grease can be skimmed off the top to reuse acetone. Drawbacks include volatility, explosiveness, availability, disposal concerns and higher cost.
Benzyl Alcohol Low toxicity, very polar in water but quite expensive.
Chloroform Great degreaser. Not typically sold at stores. Nonpolar solvent. Likely a restricted substance.
Cyclohexane Fantastic degreaser. Non-polar. Expensive, Explosive, flammable and volatile. Do not heat. Products with this are likely available.
d-Limonene Common natural terpene derived from citrus. Non-polar solvent. Not water miscible.
Ethanol Excellent degreaser of bone, highly polar solvent, but may cause staining and evaporates quickly.
Ethyl Lactate Solvent used in Bare Bones Solutions degreaser. Found in wine (Sharma et al, 2025), this green solvent (Dolzhenko, 2020) can biodegrade in carbon dioxide and water (Kua et al, 2014). This solvent is miscible in water and is amphiphilic, meaning it is both lipo- and hydrophilic. It is effective at dissolving both polar and non-polar substances, and works effectively on triglycerides.
Gasoline Strong degreaser that is readily available. Has some non-polar solvents. Drawbacks include volatility, high cost, flammability and explosiveness, lingering smell on the skull and disposal concerns.
Glycol Ethers Group of strong solvent degreasers which was tested by Bare Bones Solutions. Can be polar or non-polar. Some disposal and handling concerns because many are toxic. When considering use, propylene glycol is favorable for toxicity and ecological reasons. Propylene glycol is common in laundry detergents and is highly polar, making it easily miscible in water. Effective at miniscule concentrations of less than 0.5-1% and compatible with many surfactants and emulsifiers. They are known to be effective at cleaning bone (Stemmer and Kehagias, 2018). Glycol may induce warping and other damage in bone. In an experimental mix which included glycol, ostrich skull elements warped and a pig skull became powdery.
Heptane Non-polar. Much success has been found using Heptane, having removed 30% of fats in just 21 days (Guilminot et al, 2014). Expensive, volatile, not miscible in water and toxic. Not recommended outside of proper laboratories.
Hexane Non-polar. Very hard to source, very expensive with disposal issues. Recommended only for the highest priority and rarest specimens. Not recommended for casual use outside of a museum or laboratory.
Sulfolane Toxic and highly polar. Not suggested outside of a laboratory setting.
T-butyl Alcohol Non-polar. Probably a decent degreaser of bone. Miscible in water but expensive.
Toluene Non-polar. Decent degreaser of bone. Available in lacquer thinner. Very flammable, expensive and has health risks. Not recommended.
Turpentine Low polarity. Moderate degreaser. Easy to source, price escalates fast, noxious. Not the best choice, but could be useful for smaller skulls if nothing else is available.
Enzymes
Enzymes are catalysts, often proteins, that speed up chemical reactions in organisms. They are successful in some bone preparation (Boyde and Firth, 2004, Bromage et al, 2011 and Irlicht et al, 2002). However, “using enzymatic detergent could be considered risky to collagen in bone” (pers. comm., Wallace, J.*). Detergents contain lipases, amylases and proteases which last approximately 18 hours in solution (pers. comm., detergent company^). A commonly used protease is subtilisin (Maurer, 2004). “Nearly ten” from this family of enzymes are known to damage type 1 collagen (Ran et al 2013). Piazza and Garcia (2014) and Fujimoto (1968) point out that the triple helix of collagen prevents or slow proteolysis. The rate of proteolysis was greater in subtilisins than trypsin (Piazza and Garcia, 2014). Their tests were just 48 hours and despite some of the collagen being derived from bone it was ground in meal and not in situ, creating a discrepancy between their results and degreasing whole bone which requires much longer exposure. Most other enzymes have great difficulty interacting with collagen.
Pronase, a non-specific protease used in some detergents, may also damage collagen rich in hydroxyproline, such as type 1 (Fujimoto, 1968) at thermal thresholds around 35 degrees Celsius. Therefore, if using an enzymatic detergent, a lower temperature may be ideal.
Trypsin is an enzyme used in diaphanization. Thomas and Seymour-Jones (1923) demonstrated that it causes “measurable hydrolysis of the collagen” and Liu et al (2018) claim that “collagen fibres were partially degraded into collagen fibrils by trypsin”. Burns and Meadow (2013) report success preparing bone with trypsin when paired with enzyme detergents.
Bromelin, papain and other fruit derived enzymes, have also been used. These are added often during maceration. These likely cannot interact negatively with collagen.
*Joseph Wallace. Bone Biology and Mechanics Lab, Weldon School of Biomedical Engineering, Purdue University. Email to author, February 15, 2025.
^Multinational detergent company. Phone call with author, April 11, 2024.
Other Techniques
Maceration is a useful technique in removing grease from greasy bone. Typically this is done in 30-35 degrees Celsius water, with water changes occurring every 48 or so hours. Cold water applications are successful as well and reportedly much less odorous, but take significantly longer. This should be done ahead of chemical application.
Drilling small holes in tooth sockets, in particular in the jaws and near the zygomatic, can aid in letting grease out and degreaser in to the denser dentary bone. This is potentially favorable because any damage by the drill is covered by replacing the tooth in the socket.
Light has had some success in degreasing (Horak et al, 2022). Their study found that macerated bone achieved a desired condition of degreasing in 21 days in sunlight. Several other forms of light were used, and all appear to have had some success. It is the suspicion of this author that these bones were not only not fully degreased to standard, but that the UV light from the sun damaged collagen.
EDTA or Ethylenediamine Tetraacetic Acid is used in some cleaners to remove blood or metal stains. As a chelator, this is also used to decalcify bone (Alers et al, 1999). It is suggested that this chemical and other strong chelators included be avoided.
Vitamin C or Calcium Ascorbate is a chelating agent and is used as a dechlorinator in pools. It may help neutralize chlorine in bones treated with bleach and prevent further damage. Calcium ascorbate is the salt form buffered with calcium, which should make it less damaging compared to ascorbic acid (Vitamin C). Organic acids are used to remove blood stains, so ascorbic acid may have other uses in skull cleaning. Neither application has been tested, but both are hypothetically reasonable. May damage the hydroxyapatite with enough dosage, therefore testing and caution is suggested.
Adipocere
Formation This waxy substance has been studied for over two centuries, and presents novel taphonomy and serious obstacles when skeletonizing dead animals. This material is stable and resilient, often more than collagen and fat, and therefore requires special consideration. It consists of entirely saponified specific fatty acids (O’Brien and Kuehner, 2007 and Ubelaker and Zarenko, 2011) which may require high pH environments under specific conditions to form (Vass, 2001). The ideal pH for formation may be lower than high end degreasers, but still mildly alkaline (Ubelaker, 2023), and require anaerobic conditions at 21-45C for optimal enzymatic release and bacterial growth (Forbes et al, 2011). Schoenen and Schoenen (2013) report that a lack of both oxygen and “bacterial degradation” is key to adipocere formation. Excellent summaries can be found on google scholar in Forbes et al, 2004, Takatori, 2001, Ubelaker and Zerenko, 2011 and others.
Magni et al (2021) makes a distinction between saponification and adipocere formation, and describes the chemical process in detail. They also indicate Bacillus subtilis, Micrococcus luteus, Staphylococcus aureus, some Pseudomonas species and Clostridium perfringens may be involved. B. subtilis generates subtilisin enzymes for cleaners, and C. perfringens can survive in higher pH environments and has a varied diet but prefers glucose. It may be possible to limit adipocere growth by controlling bacterial food sources or growth. This is currently being investigated.
Glucose may be important in adipocere formation because of its role as bacterial food and presence in bone marrow. There may be a different glucose level in marrow than ambient blood levels (Suchacki et al, 2020) due to varying “insulin responsiveness” in different kinds of marrow tissues. Varying diets, such as those in canids and ursids compared to others in the carnivora, should cause the composition of bone marrow tissues to differ (Dzubanova et al, 2024). This may explain the observed tendency of canids and ursids to develop adipocere compared to other canids.
Removal There have been successful reports of some products removing adipocere (Obermeyer et al, 2011). It seems likely that any sufficiently powerful degreasing agent may remove adipocere well. Adipocere naturally forms in high pH environments through saponification. Ammonia is indicated as a remover of soap scum, and may function as a final wash to clean up adipocere residue formed during the process.
The melting point of adipocere is reported as 71 C by Ruttan and Marshall (1917). Melting it without damaging collagen may not be possible because the uppermost heat breakpoint of collagen may only be as high, at maximum, as approximately 61 degrees Celsius (Danielsen et al, 1994, reported shrinking in human pelvises at that temperature). It is well known in art communities that oil melts wax. With a very small amount of olive oil (triglyceride) and a stiff bristle brush, the majority of adipocere was successfully removed from a canid skull in a test. This miniscule amount of oil (less than half of one teaspoon) mostly washed away with soapy water, and likely would degrease away easily.
Grease Crystallization
There is a belief that heating grease will cause it to move “deeper into the bone” and make it harder to degrease. That grease can be moved through dense cortical bone indicates this hypothesis may be incorrect because “deeper grease” should still be movable through bone. An alternative hypothesis is that the triglycerides are crystalizing post-heating. According to Gunjan et al., 2024, slow-cooled triglycerides required ten times the amount of time to degrease successfully with a surfactant. Bone is a decent insulator, and any grease inside it may cool slower as a result. When they cool slower, the crystals are bigger and the lattice is more organized, making it harder to degrease.
Tristearin, a long-chain (saturated) triglyceride, has three or four plateaus as heat changes where crystallization can occur (Hoerr and Harwood, 1956). The lowermost melting point of this triglyceride is 55 degrees Celsius, which exceeds collagen’s comfort temperature. When excessive heat is applied, these crystallization plateaus may be reached. In highly polar solvents, solubility of tristearin limited. In non-polar solvents, solubility is considerably higher. These observations are consistent with grease behavior reported by skull preparators when dealing with skulls with an excessive thermal history. This author hypothesizes that non-polar solvents will likely be more successful than surfactants in handling most crystallized grease.
Conclusion
Bone degreasing has many options and there is no silver bullet or universal technique. Online resources are disparate, discombobulated, amateur and largely unreferenced. It is the hope that this information may remedy some of these issues and begin to organize some of the information in a more formal and accessible way. Please reach out if there are errors.
References
Alers, J.C., Krijtenburg, P.J., Vissers, K.J. and van Dekken, H. (1999). Effect of bone decalcification procedures on DNA in situ hybridization and comparative genomic hybridization. EDTA is highly preferable to a routinely used acid decalcifier. Journal of Histochemistry and Cytochemistry. 47(5), 703-10. doi: 10.1177/002215549904700512
Boman, A. and Maibach, H.I. (2000). Percutaneous absorption of organic solvents. Int J Occup Environ Health, 6(2), 93-5. doi: 10.1179/oeh.2000.6.2.93
Bosch, P., Aleman, A., Moreno-Castilla, C., and Botella, M. (2011). Boiled versus unboiled: a study on neolithic and contemporary human bones. Journal of Archaeological Science. 38(10), 2561-2570. doi: 10.1016/j.jas.2011.04.019
Boyde, A. and Firth, E.C. (2004). Articular calcified cartilage canals in the third metacarpal bone of 2-year-old thoroughbred racehorses. Journal of Anatomy, 205(6), 491-500. doi: 10.1111/j.0021-8782.2004.00354
Bromage, T.G., Juwayeyi, Y.M., Smolyar, I, Hu, B, Gomez, S, Chisi, J. (2011). Enamel-calibrated lamellar bone reveals long period growth rate variability in humans. Cells Tissues Organs, 194(2-4), 124-30. doi: 10.1159/000324216
Burns, P. and Meadow, R.H. (2013). The use of trypsin to prepare skeletal material for comparative collections with a focus on fish. Archaeofauna, 22. 29-36
Burton, B., Gaspar, A., Josey, D., Tupy, J., Grynpas, M. D., And Willett, T. L. (2014). Bone embrittlement and collagen modifications due to high-dose gamma-irradiation sterilization. Bone. 61, 71-81. doi: 10.1016/j.bone.2014.01.006
Danielsen, C.C., Mosekilde, L., Bollerslev, J., and Mosekilde, L. (1994). Thermal stability of cortical bone collagen in relation to age in normal individuals and in individuals with osteopetrosis. Bone, 15(1), 91–96. doi: 10.1016/8756-3282(94)90897-4
Dolzhenko, Anton. (2020). Ethyl lactate and its aqueous solutions as a sustainable media for organic synthesis. Sustainable Chemistry and Pharmacy, 18. doi: 10.1016/j.scp.2020.100322
Dzubanova, M., Benova, A., Ferencakova, M., Coupeau, R. and Tencerova, M. (2024). Nutrition and bone marrow adiposity in relation to bone heath. Physiological Research. 73(1). 107-138. doi: 10.33549/physiolres.935293
Eriksen, E.F., Charles, P., Melsen, F., Mosekilde, L., Risteli, L., and Risteli, J. (2009). Serum markers of type I collagen formation and degradation in metabolic bone disease: Correlation with bone histomorphometry. Journal of Bone and Mineral Research, 8(2), 127–132. doi:10.1002/jbmr.5650080202
Forbes, S.L., Stuart, B., Dadour, I., and Dent, B. (2004). A Preliminary Investigation of the Stages of Adipocere Formation. ASTM International. J. Forensic Sci., 49(3). doi: 10.1520/JFS2002230
Forbes, S.L., Wilson, M.E. and Stuart B.H. (2011). Examination of adipocere formation in a cold water environment. Int J Legal Med. 125(5):643-50. doi: 10.1007/s00414-010-0460-6
Fujimoto, D. (1968). Correlation between pronase susceptibility and hydroxy proline content of collagen fiber. Biochimica et Biophyica Acta (BBA) - Protein Structure. 154(1); 22, 183-189
Guilminot, E., Lemoine, G., Pelé, G., Poisson, L., Surbled, M., Louvet, I., Mevellec, J.-Y., and Rémy, L. (2014). Re-treatment of whale bones – How to extract degraded fats from weakened bones? Journal of Cultural Heritage, 15(2), 128-135. doi: 10.1016/j.culher.2013.03.008
Gunjan, T., Torquato, L. M. G., Ahmad, Z., Fong, R., and Cabral, J. T. (2024). How triaclyglycerol thermal history impacts film removal by surfactant solution. Journal of Colloid and Interface Science, 670 (15), 540-549. doi: 10.1016/j/jcis.2024.05.069
Horak, O., Pyszko, M., Paral, M., and Sandor, O. (2022). Degreasing and bleaching bones using light sources as a tool to increase the safety of teaching osteology at the university of veterinary science, Brno. Peer J. doi: 10.7717/peerj.14036
Hoerr, C.W and Harwood, H.J. (1956). The solubility of tristearin in organic solvents. The Journal of Physical Chemistry. 60(9), 1265-1269. doi: 10.1021/j150543a028
Irlicht, R.A, O’Connell, S.L., Thomas, C.D.L. and Clement, J.G. (2002). The effect of Terg-a-zyme on the breaking strength of bone. ANZBMS 12th Annual Scientific Meeting. Stamford Grand, Adelaide, Australia.
Jantschke, A., Spinde, K. and Brunner, E. (2014). Electrostatic interplay: The interaction triangle of polyamines, silicic acid, and phosphate studied through turbidity measurements, silicomolybdic acid test, and 29 Si NMR spectroscopy. Beilstein journal of nanotechnology, 5, 2026-35. doi: 10.3762/bjnano.5.211
Kua, Y.L., Gan, S., Ng, H.K. and Morris, A. (2014). The potential of ethyl lactate as a green solvent to extract carotenoids and vitamin e from crude palm oil. Proceedings of the ISPFVF University of Nottingham Malyasia Campus, Semenyih, Malaysia: 11-13
Lambri, L.L., Bozzano, P. B., Giordano, E. D. V., Bonifacich, F. G., Gargicevich, D., Zelada, G. I. and Lambri, O. A. (2018). Denaturation processes of collagen from cow bones as a function of temperature. Materia, Rio J, 20(2)
Leikina, E., Mertts, M.V., Kuznetsova, and Leikin, S. (2002). Type 1 collagen is thermally unstable at body temperature. Biophysics and Computational Biology. 99(3), 1314-1318. doi: 10.1073/pnas.032307099
Liu, Z-q., Tuo, F-y., Song, L., Liu, Y-x., Dong, X-p., Li, D-m., Zhou, D-y. and Shahidi, F. (2018). Action of trypsin on structural changes of collagen fibres from sea cucumber (Stichopus japonicus). Food Chemistry. doi: 10.1016/j.foodchem.2018.02.117
Maurer, K-H. (2004). Detergent proteases. Current Opinion in Biotechnology. 15(4), 330-334. doi: 10.1016/j/copbio.2004.06.005
Magni, P.A., Lawn, J. and Guareshi, E. E. (2021). A practical review of adipocere: key findings, case studies and operational considerations from crime scene autopsy. Journal of Forensic and Legal Medicine. 78. doi: 10.1016/j.jflm.2020.102109
Nandi, P. K., Grant, M. E. and Robinson, D. R. (1985). Destabilization of collagen structure by amides and detergents in solution. International Journal of Peptide and Protein Research. 25(2), 206-12. doi: 10.1111/j.1399-3011.1985.tb02166.x
O’Brien, T.G. and Kuehner, A.C. (2007). Waxing grave about adipocere: soft tissue change in an aquatic context. J. Forensic Sci. 52, 294–301
Obermeyer, C., Finnegan, F. and Salem. S. (2011). Adipocere Removal from Human Skeletal Remains. Poster, Chemistry Department, Washburn University, Topeka, Kansas
Piazza, G.J., and Garcia, R. A. (2014). Proteolysis of meat and bone meal to increase utilisation. Animal production Science. 54, 200-206. doi: 10.1071/AN13041
Ran, L.-Y., Su, H.-N., Zhao, G.-Y., Gao, X., Zhou, M.-Y., Wang, P., Zhao, H.-L., Xie, B.-B., Zhang, X.,Y., Chen, X.-L., Zhou, B.-C., and Zhang, Y.-Z. (2013). Structural and mechanistic insights into collagen degradation by a bacterial collagenolytic serine protease in the substilisin family. Molecular Microbiology. 90(5), 997-110. doi: 10.1111/mmi.12412
Ruttan, R.F. and Marshall, M.J. (1917). The composition of adipocere. The Journal of Biological Chemistry. 29(2), 319-327
Schoenen, D., and Schoenen, H. (2013). Adipocere formation- The result of insufficient microbial degradation. Forensic Science International. 226 (1-3). doi: 10.1016/j/forsciint.2013.01.023
Sharma, C., Bakshi, A., Kharka, S. and Sharma, M. (2025). Blending of a wine congener (ethyl lactate) with some fatty acid ethyl esters (ethyl caprate, ethyl laurate, and ethyl myristate): volumetric, transport and acoustic approach. Journal of Chemical & Engineering Data. Article ASAP doi: 10.1021/acs.jced.4c00467
Shehata, T.P. and Krap, T. (2024). An overview of the heat-induced changes of the chemical composition of bone from fresh to calcined. International Journal of Legal Medicine, 138, 1039-1053. doi: 10.1007/s00414-024-03160-z
Stemmer, D. and Kehagias, O. (2018). Finding a new solution to an old problem. Biodiversity Information and Science Standards. Sofia. doi: 10.3897/biss2.26392
Suchacki, K.J., Tavares, A.A.S., Mattiucci, D., Scheller, E.L., Papanastasiou, G., Gray, G., Sinton, M.C., Ramage, L.E., McDougald, W.A., Lovdel, A., Sulston, R.J., Thomas, B.J., Nicholson, B.M., Drake, A.J., Alcaide-Corral, C.J., Said, D., Poloni, A., Cinti, S., Macpherson, G.J., Dweck, M.R., Andrews, J.P.M., Williams, M.C., Wallace, R.J., van Beek, E.J.R., MacDougald, O.A., Morton, N.M., Stimson, R.H. and Cawthorn, W.P. (2020). Bone marrow adipose tissue is a unique adipose subtype with distinct roles in glucose homeostasis. Nature Communications. 11(3097). doi: 10.1038/s41467-020-16878-2
Takatori, T. (2001). The mechanism of human adipocere formation. Legal Medicine. 3(4), 193-204. doi: 10.1016/S1344-6223(01)00036-0
Thomas A.W. and Seymour-Jones F.L. (1923). The hydrolysis of collagen by trypsin. Proceedings of the Society for Experimental Biology and Medicine. 20(8), 433-434. doi:10.3181/00379727-20-215
Turner-Walker, G. (2012). The removal of fatty residues from a collection of historic whale skeletons in Bergen: an aqueous approach to degreasing. doi: 10.13140/2.1.1996.8969
Ubelaker, D.H. and Zarenko, K.M. (2011). Adipocere: what is known after over two centuries of research? Forensic Science International. 208(1-3), 167-172. doi: 10.1016/j.forsciint.2010.11.024
Ubelaker, D.H. (2023). Postmortem inteverval. Encyclopedia of forensic sciences, third edition. Ed M. M. Houck. Elsevier. 241-245. doi.org/10.1016/B978-0-12-823677-2.00003-9
Van Heiningen, A.R.P., Kang, G.J. and Ni. Y. (1999). Addition of sodium silicate and chelant to the FAS stage to blach recycled fibers. Pupling Conference Proceedings.,
Vass, A.A. (2001). Beyond the grave – understanding human decomposition. Microbiol. Today. 28, 190-192.
Vere, K-A., Richens, J.L., Lane, J. S., Harris, H.J., Duggan, J. and O’Shea, P. (2013). Evidence for sodium metasilicate receptors on the human osteoblast cell surface; spatial localization properties. Molecular Membrane Biology. 30(8), 386-393. doi: 10.3109/09687688.2013.843031
Viguet-Carrin, S., Garnero, P., and Delmas, P. D. (2005). The role of collagen in bone strength. Osteoporosis international. 17(3), 319-336. doi: 10.1007/soo198-005-2035-9
White, E.M., Hannus, L.A. (1983) Chemical weathering of bone in archaeological soils. American Antiquity. 48, 316-322.
Zdzieblik, D., Oesser, S. and König, D. (2021). Specific bioactive collagen peptides in osteopenia and osteoporosis: long-term observation in postmenopausal women. Journal of Bone Metabolism. 28(3), 207-213. doi: 10.11005/jbm.2021.28.3.207.
Zhang, Y.J., Kang, L. and Liu, L.C. (2015). Alkali-activated cements for photocatalytic degradation of organic dyes. In: Handbook of Alkali-Activated Cements, Mortars and Concretes. Eds F. Pacheco-Torgal, J.A. Labrincha, C. Leonelli, A. Palomo and P. Chindaprasirt. Woodhead Publishing. 729-779 doi: 10.1016/C2013-0-16511-7
Web version 1.092
© 2025 Steve Kary
changelog
-June 17 added Leikina et al 2002 and collagen stuff to heat section. Added some defintions to body text.
-June 11 added vitamin C
-May 29 added some new specific info to the heat section and 2 new refs
-May 21 fixed up bone types wording (Thanks Jonathan Aghion!)
-April 19 added Hoerr and Harwood 1956, updated crystallizations section and fixed an error saying polar solvents would work better when non-polar should be ideal.
-April 3 minor update to metasilicate and update to lauryl sulfate (surfactant) section
-March 24 updated adipocere section
-Feb 20 added small section about EDTA
-Feb 17 enzyme section updates, truncated changelog
-feb 15 enzyme section updates
FAQs
-
Sodium Metasilicate is the saponifier and Ethyl Lactate is the solvent.
-
Yes! Ethyl Lactate is listed by several authors as a green solvent, and Sodium Metasilicate is generally considered to be eco-friendly.
-
In solution it has a mild soapy odor unless it has a large grease load.
-
Progress in this project has been managed in phases. Release schedule will be no different and dependent on local and national growth, as well as other factors. It is something that has been discussed, but the immediate focus has been Canadian release.